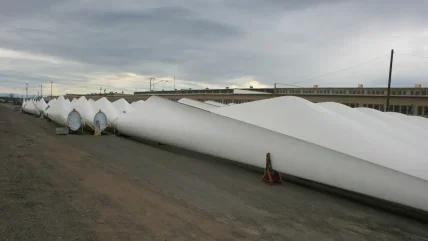
Blade design is arguably the most important aspect of turbine manufacture, with a direct bearing on how much energy is produced and how much it costs. As designs become more innovative, testing procedures need to become more meticulous. Kevin Standish, R&D LAC engineering manager at Siemens Wind Power, explains how the testing regime feeds back into the manufacturing process, in the laboratory and on the field.
For any wind turbine manufacturer, the blades are undoubtedly the most heavily scrutinised component of the design. While the tower and the nacelle are subject to their own design challenges too, it is the blades where the real experimentation occurs.
Which materials should be used? How can the blade weight be optimised and its longevity increased? Since the blade contributes most to the cost of energy, it stands to reason that this should be the key point of focus.
Recent years have seen a considerable amount of innovation in blade design, typically geared around making the turbine as economical and eco-friendly as possible. In practice, this often means increasing the size of a turbine.
A number of companies are currently working on the development of a 10MW turbine, with existing examples such as the 8MW Vestas V164 setting the bar for what can be accomplished. Siemens, meanwhile, has designed a 6MW offshore prototype with 75m blades.
Even when a turbine is not set to break any size records, designers must perform a carefully calibrated balancing act, geared around keeping costs down. They need to make the blade as light as possible, in a view to save material and operational expenses, without sacrificing its strength or power-generation capabilities. This means taking an exploratory, open-minded approach.
"The cost of energy is calculated by looking at the amount of energy produced divided by the total cost. The rotor produces 100% of the energy, so if you can come up with innovative, new technologies or blade design features, you are directly impacting the cost of wind power," explains Kevin Standish, R&D LAC engineering manager at Siemens Wind Power.
What this means in practice is implementing a meticulous testing regime. Arguably, the more novel the blade design, the greater the scope for something to go wrong. Testing needs to occur every step of the way – in the laboratory and the field – with the results seamlessly feeding back into the manufacturing process.
"At Siemens, design, testing and manufacturing go hand in hand," says Standish. "It’s our job to test the blades and to ensure that they are safe, high quality and efficient. In our wind R&D centre in Boulder, Colorado, US, we are constantly working to strengthen our blade technology and quality for the global market.
"With all of the sensors that are on the blades during testing, we can see potential issues as they occur. If we notice an issue emerge, we immediately stop testing to investigate and determine a solution. The results of our tests, and any potential design implications, are then used to manufacture the final product."
Safety check
The testing process begins even before blades are designed, with extensive materials testing. Particularly as carbon-fibre composites become more popular, replacing glass, it is critical to ensure that any new material choices are defect-free and that the proposed fibres are compatible with the proposed resin. Siemens performs a large proportion of this testing in house, in order to ensure optimal structural material properties.
Once blade design commences, the first step is to ensure the blade is structurally sound. This is achieved via static structural testing, which is carried out on the flap-wise and edge-wise orientation of the blades. The idea is to apply an extreme load profile (up to 110% of maximum load), and pull it in both directions to see how it deflects. This enables the blade to receive its prototype certificate.
"When the blade has completed its static testing and is structurally sound, we can fly it on a turbine and put it through fatigue testing," explains Standish. "This process is designed to test a blade through any conditions it may encounter in a 20-year life span. In order to receive a provisional certificate, the blade must be able to survive 10% of fatigue life. After the completion of fatigue testing, you do static testing once more to receive the final certificate and ultimately begin mass production."
Since 2001, wind manufacturers across the globe have been beholden to the IEC 61400 standard, created by the International Electrotechnical Commission. This standard concerns safety, measurement techniques and test procedures for wind turbine generator systems. In the case of offshore turbines, a number of additional standards may also come into play.
Because different blades will be subject to different load levels, it’s important to ascertain the wind climate of the proposed turbine
site. The IEC’s requirements vary depending on turbine class, which is in itself determined by a complex mix of factors: average wind speed, turbulence and extreme 50-year gust (the wind speed you would expect, algorithmically, to occur just once in half a century).
The outside edge
Aside from the bare minimum required to obtain certification, manufacturers may wish to perform a number of additional tests, giving them the edge in an increasingly competitive market.
"At Siemens, we also perform extensive tests that may not be required for certification, ranging from wind tunnel tests and lightning tests to accelerated erosion testing, to ensure that our turbines are the highest quality and can safely endure any conditions that may be present in the field," says Standish.
While Siemens has its own blade testing facilities – and therefore performs 100% of its testing in house – smaller companies may need to go further afield. In the US, the National Renewable Energy Laboratory (NREL) provides highly versatile facilities, offering research and analysis of wind turbine components and prototypes rated from 400W to 5MW. These capabilities, based at the National Wind Technology Center in Boulder, Colarado, enable structural testing, drivetrain testing and field-testing for turbine manufacturers of any size.
The site has a strong track record in developing innovative test systems, geared around improving accuracy and decreased test duration. For instance, it offers a controllable grid interface test system, which can simulate faults and provides a controlled laboratory environment. It also developed the Universal Resonant Excitation system, which has since been commercialised
as an industrial product.
As blades get longer and material choices change, testing standards will need to reflect a very different suite of challenges. As Standish explains, earlier forms of static testing are simply not sufficient for the newer generations
of turbine models.
"One of the biggest changes lately is the nature of the blades themselves," he says. "Today, blades are much more aeroelastic – made with composite materials like fibreglass that allow them to flex, and reduce weights and loads. These are elements that we incorporated in a new turbine, the SWT-2.3-120, that we recently introduced specifically for the Americas region.
"When you test these blades, you test in flap and edge directions. But in the real world, forces that may impact the blade are not purely in these two directions. These in-between loads are referred to as ‘sweep loads’. Looking forward, this type of off-axis extreme loading is now beginning to become incorporated into testing and standards. Including a broader scope of design load cases that could potentially lead to failure ensures that a blade can maintain its integrity for those cases as well."
Wearing times
Another challenge to emerge in recent years has been that of blade leading-edge erosion, which has been flagged up as a high-priority concern by the offshore industry. Erosion reduces turbine aerodynamic performance and structural integrity, with direct effects on energy capture. And while there are solutions in place – high solids tape and protective tape – these repairs don’t always last for long, which dramatically hikes up costs.
A joint industry project, BLEEP, has therefore been set in motion, to develop test facilities for advanced leading-edge protection at sea. In this scenario, and many others, the industry is best served by joining forces with others in the know.
"At Siemens, we believe that collaboration is critical to accelerating technology development," says Standish. "Academia and research institutions are instrumental in bringing forward and proposing new ideas but lack the real-world experience of the OEMs. By working closely together, Siemens can help further direct and mature the most promising technologies with the ultimate goal of integrating them into our products."
With any wind turbine, the bottom line is that it has to last a while. Testing regimes therefore need to be extremely thorough, keeping
an eye on any future difficulties that might arise. And as blade design moves with the times, testing will need to follow suit.
"We simulate for every scenario imaginable that could occur over the 20-year lifespan of the turbine," explains Standish. "This wide range of potential scenarios forms the design load envelope that defines the levels to which we test."